Just months after opening its doors, the Kendeda Building for Innovative Sustainable Design is on the cusp of its drinking water permit.
That’s a key threshold for any large Living Building Challenge project, because drinking water permits present one of the most difficult hurdles for LBC certification. Like most other Living Building projects, however, the Kendeda Building’s treatment won’t be free from the use of chlorine — a chemical that the stringent certification system discourages.
The LBC requires buildings to provide their own water for faucets and fountains by capturing water (usually via rainfall), treating it without the use of potentially harmful chemicals, and then treating it again after use, before finally discharging it onsite. The idea of the “Net Positive Water Imperative” is to replicate the hydrology of natural systems in the way that they enrich the soil, recharge groundwater and reduce the frequency of flooding.
Rather than being handled strictly as an energy-intensive resource to be diverted, chemically treated, and pumped through a series of pipes before it’s used, then sent through more pipes, treated again and discharged, drinking water is restored to its role in hydrological cycle: It falls from the sky, is used by one species (us), and then used by plants and animals as it gradually flows into natural waterways. At least, that’s the idea.
Breaking new ground at the building scale
In the United States, potable water treatment has proven the trickiest part of the imperative for large, publicly accessible buildings because, under the federal Safe Drinking Water Act, any system that serves more than 25 people a day must be permitted as if it were a municipal water works.
“They’re not used to somebody coming along and saying, ‘We want to get a drinking water permit for one building,’” said John J. DuConge, the Georgia Tech senior project manager who oversaw construction of the Kendeda Building.
Mark Azarraga, a plumbing engineer at Newcomb-Boyd in Atlanta, helped to design the Kendeda Building filtration and treatment system. In an email, he described it this way:
While the majority of the potable water treatment occurs in the basement level of the Kendeda Building, water treatment actually begins inside the rainwater pre-filters that are visible at two locations on the building’s main level. The pre-filters remove large solids (leaves, etc.) from the rainwater that is collected from roof areas – the filtered rainwater is then stored in the building’s internal cistern that is located on the basement level.
As the pre-filtered rainwater is drawn from the cistern via the transfer pumps, it is first routed through multiple stages of filtration which further reduces the suspended particulate counts and improves the water’s turbidity (measure of water’s transparency). What follows is a dose of ultraviolet sterilization to eliminate bacteria, viruses, spores, organisms, etc.
After UV treatment the water passes through a chlorine “dosing station.” But, following the guidelines of the LBC, Georgia Tech initially hoped never to have to rely on chlorine, which was included only as a backup.
It was unusual enough for a single building to seek its own drinking water permit. But proposing to treat the water without chlorine was downright eccentric.
“It’s uncommon, it hasn’t been done at this scale in the state of Georgia, and there’s no regulatory existing pathway or unique path for this project to get a permit,” Erin English, senior engineer at Biohabitats Inc. and a veteran of other LBC projects, said in 2017 as she and her colleagues developed the overall scheme for how the Kendeda Building would handle water. “But this project has benefited from a lot of us banging our heads against the wall on previous projects.”
Earlier projects lay the groundwork
It was far from the first time that the LBC’s regenerative approach to water treatment would run into practical and regulatory challenges. Famously, it took the landmark Bullitt Center five-and-a-half years to receive its drinking water permit and to switch from city water to rainwater. More recently, the Kern Center at Hampshire College in Massachusetts was only able to obtain its drinking water permit two years after it opened, but that building has repeatedly had to switch back and forth between its own system and city water.
English advised Georgia Tech to lay the groundwork for the drinking water permit as early as possible. So even before construction began, Nazia Zakir, the university’s assistant vice president for environmental health and safety, engaged officials at the state Environmental Protection Division in a dialogue. This helped Zakir to identify potential issues that had to be sorted through before obtaining the permit.
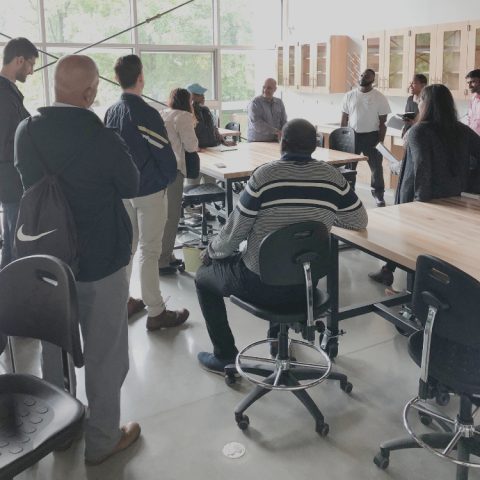
“We were skeptical if it was going to work. But we were on board to support the effort,” EPD Drinking Water Program Manager Manny Patel wrote to me later in an email. “To the best of my knowledge this is the only building in the state that has asked permission to use rainwater in lieu of city water as the source of drinking water.”
It certainly didn’t hurt that Georgia Tech was a fellow state institution. Or that — as the state’s flagship engineering school — the university had positioned the project as a living laboratory for innovative sustainable design. Or, for that matter, that Zakir led a staff that regularly deals with permitting issues — a resource that privately owned buildings and smaller institutions don’t have. Still, even for Georgia Tech, the path toward a permit has been challenging.
Among the first actions Zakir and her team needed to take to satisfy state officials was to set up a mock system using solar panels on the roof of existing building, together with a bucket to collect rainwater.
“We were asked to capture samples from three rain events,” she said at the time. “It’s taken some time. We have to wait to put the rainwater collection bucket out for significant rain, so that it’s not accumulating a lot of dirt and leaves and stuff [when it’s not raining].”
Zakir then had to engage an independent lab to test the runoff and to demonstrate to the EPD that it wouldn’t pick up pollutants before being treated for potable use inside the building.
EPD also required that the building include a connection to the municipal water system so that city water could be used in case the building’s own system wasn’t meeting federal drinking water standards. That connection was important when it became clear that water going through the building’s treatment system couldn’t be tested before the building opened in September.
30 days of real-world testing
Since opening, the building has for the most part been using city water. The exception was a 30-day period in the fall during which the university brought temporary sinks and drinking-water stations in so that the building’s UV treatment system could be operated and tested.
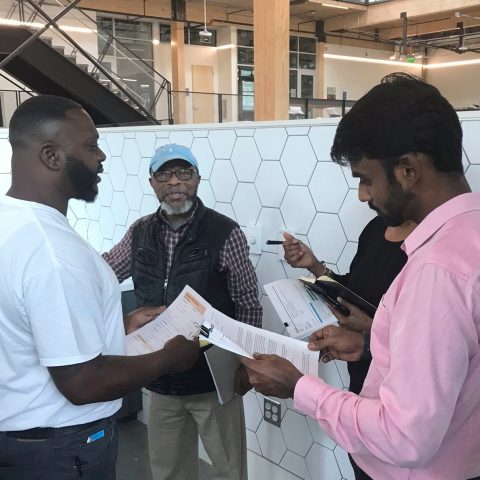
It was an arduous process,in part because there are few independent labs established to do the extensive testing required under the federal Safe Drinking Water Act. The testing schedule, which was developed by Jacobs Engineering, was extensive: Samples were collected of the water in its raw stage, after it was filtered, before it went into the distribution pipes, and at two sinks and two fountains. All told, there were samples run for a wide range of viruses, eight types of bacteria, four radio-nuclides, 28 inorganic chemicals, 88 organic chemicals, and four physical properties, such as temperature and alkalinity.
“Based on the results of the monitoring,” Jacobs project manager David Bell finally reported in a Jan. 30 letter to the state, “all parameters were in compliance with Primary and Secondary drinking water standards.” Except one. The heterotrophic plate count — a way of measuring whether a broad class of bacteria that relies on organic material could grow in the water — far exceeded the standard level of 500 “colony-forming units” per mili-liter at two points in the system: in the post-treatment cistern and after it came out of the building’s faucets.
”That had to do with the fact that we were not using chlorine,” Georgia Tech’s Zakir says bluntly.
Not surprisingly, the EPD responded by requiring Georgia Tech to activate the chlorine-dosing station.
Eliminating chlorine remains a holy grail
It’s an open question whether regulators in the United States will ever get comfortable with treatment systems that don’t include disinfection of drinking water with chlorine. Among Living Building Challenge projects serving at least 25 people daily, only the Kern Center in Massachusetts has obtained a permit not requiring chlorine. But that building has had to switch back to public water while its owner, Hampshire College, tries to figure out why the HPC levels occasionally spike.
The International Living Future Institute, which administers LBC, has basically acknowledged the difficulty by creating an exception that allows “the minimum amount allowed by the code” to be added “if required by the authority having jurisdiction.”
Internationally, there’s strong disagreement between those who advocate chlorinated drinking water and those who warn against it. In the United States, the chemical’s use is so pervasive that it’s difficult to tell what water safety would be like without it.
Researchers have tied chlorine in drinking water to higher incidences of bladder, breast and rectal cancers. At least one paper in the journal Science indicated that drinking water can be treated safely without the use of chlorine. A handful of countries, including the Netherlands, have even barred chlorine in drinking water without suffering an uptick in waterborne diseases, according to the study.
Some scientists and engineers argue that the HPC test is a faulty barometer for water safety. The test requires heterotrophic bacteria to be added to the water sample and allowed to grow. If the bacteria multiplies beyond the 500-unit-per-mili-liter standard, the sample fails.
The U.S. Environmental Protection Agency views HPC as an indication that harmful bacteria might grow in the water. HPC levels are likely high because the bacteria is able to feed off the “biofilm” that typically develops within distribution pipes inside the building. According to the prevailing theory, if that biofilm supports benign micro-organisms, it could support harmful microorganisms. Critics counter that bacteria wasn’t necessarily growing in the pipes and that the bacteria used in the test isn’t even harmful anyway.
It’s difficult to argue, however, with state regulators who are following EPA mandates. And, for their part, building owners might not want to take on the liability of arguing against an HPC test, and then at some point in the future finding that water problem eventually did make occupants sick.
Designing systems to keep people safe
Conventional treatment systems ensure that they’ll pass the HPC test by adding more than enough chlorine in the plant to treat the water there. That way there will be enough chlorine leftover to react with any bacteria that grows as the water runs through the pipes. In a municipal system, huge amounts of the chemical are needed to protect the water as it courses through miles of pipe before it even reaches a building. To ensure that the pipes are free of bacteria, permits typically require tap water to contain “residual chlorine” — an indication that all the bacteria has been killed off all the way through the system.
EPD was willing to allow Georgia Tech to test the system without the use of chlorine. Had the HPC test not shown elevated levels, the building might have been on a path to obtain a permit that didn’t require residual chlorine. But EPD’s Patel thought that was always a bit of a reach.
“EPD had concerns all along if UV [would] be able to meet the drinking water standards and growth of bacteria in the lines post UV,” he wrote. “UV is a point-of-use treatment and is not yet proven to be used in [a] larger system. The concern is that UV kills all the bacteria, viruses and pathogens as the water is passing through the UV. But that water sits in the pipes before it is used and bacteria will grow in the pipes.”
EPD’s chlorine mandate didn’t require changes to the treatment system’s because the system’s design always had included a chlorine tank and dosing station. The LBC exception does say, however, that if a regulator requires chlorine, “the project must include and document point-of-use dechlorination with a 0.5-micron carbon block filter or other approved dechlorination method for all potable end uses.”
So now Batchelor & Kimball Inc., the Kendeda Building’s plumbing contractor, must install the carbon filters at the building’s sink faucets, shower faucets and water fountains. And so that samples can be taken after the water’s run through the pipes but before the filters remove the chlorine, BKI must install a testing port “upstream” from each filter.
Those modifications, as well as another round of tests to prove to EPD that the system will work now that chlorine is being added, are being delayed by campus’ covid-19 lockdown.
“We did not want to take a chance that we would start the testing and then have to stop because [the lab] were asked to close,” wrote April Kelly, an environmental program manager in Zakir’s office who shepherded much of the permitting work and help to manage the operations once the permit is obtained. “Also there was some uncertainty with the staffing on campus and if we would be teleworking for a period of time.”
Was it worth the added costs?
Project team members estimate that the 50,000-gallon cistern that stores the rainwater before it’s treated, various pipes and the treatment system itself added some $350,000 to the cost of the Kendeda Building. That’s about 2 percent of the overall construction cost. In addition to that testing alone has already topped $20,000 and is expected to go over $23,000 by the time all the testing is complete.
To meet the requirements of the permit, the university also contracted with Marcus Thomas, a certified drinking water operator, as well as with lab to perform ongoing tests. Combined, those operational costs will run in the neighborhood of $75,000, says Kelly, the Georgia Tech environmental programs manager.
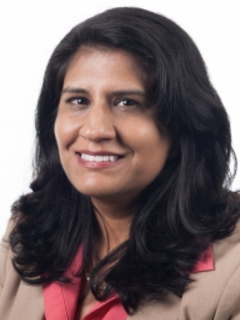
Even more than costs, however, permitting difficulties have raised questions in some quarters about the value of LBC’s approach to potable water.
“There’s an argument to be made that treating your own water onsite isn’t worth the effort,” notes Dennis Creech, fund advisor for sustainability at the Kendeda Fund (which publishes this blog). “I think that’s an open question.”
Several of those directly involved in the Georgia Tech project have grown to appreciate the potable water challenge despite its difficulties, however. Zakir — whose position requires her to be pragmatic about complying with conventional rules — is one of them. Among the advantages, she argues that future projects, particularly in Georgia, will have a better road map toward living up to the LBC’s aspirations. They also may be dealing with state regulators who are more familiar with the concepts.
“When I first heard about this project,” she says. “I was highly skeptical and now I’m very much on board with using rainwater as a potable water resource. It has turned my thinking around in the sense that it can be done. It’s very expensive but we sort of laid the groundwork.”
PHOTO AT TOP: Drinking water treatment equipment in the basement of the Kendeda Building. Photo by Ken Edelstein.
It does not surprise me EPD would put the system through multiple hoops. They are not big on rainwater. However, this link might help https://www.winespectator.com/articles/silver-oak-becomes-first-winery-to-earn-living-building-challenge-certification